Carbon utilization converts waste to worth
While carbon capture is effective in reducing greenhouse gas emissions, it is an expensive process. CO₂ utilization presents a promising solution by offsetting some costs and offering more sustainable practices across various industries

Na kratko
- Carbon utilization partially offsets the cost of carbon capture by transforming captured CO₂ into valuable products, instead of just storing it and incurring additional expenses.
- Carbon capture, utilization and storage technologies are applicable for multiple industries. Key examples include creating sustainable building materials for the construction sector, producing renewable fuels for the transportation industry, synthesizing chemicals and polymers in the chemical sector, enhancing oil recovery in the O&G industry, and boosting food security in the agricultural industry.
- Carbon utilization exemplifies a circular economy by repurposing carbon dioxide captured from waste streams.
- The high cost of carbon capture technology, the need for significant infrastructure investment and the requirement for renewable energy sources present substantial hurdles to its widespread adoption.
- Continued research and development, along with a global focus on sustainability, are expected to drive innovation, reduce carbon capture costs and pave the way for broader adoption across various industries.
Adding value
Carbon capture is an emerging answer to reduce industrial greenhouse gas emissions (GHG). After capturing carbon dioxide from process exhaust streams, it is typically transported from its origin site for long-term storage underground. However, the technology and ongoing monitoring of storage sites are costly.
To partially offset the expenses, captured CO₂ could be repurposed to create value instead of simply being stored. This practice, known as carbon recycling, falls under the broader umbrella of carbon capture, utilization and storage (CCUS), a collective solution to help meet 2050 net-zero emissions targets and mitigate climate threats.
Converting challenges into opportunities
Today, industry collects 45 million metric tons of carbon dioxide from waste exhaust streams annually, amounting to around 0.1% of global emissions from all sectors. According to climate models from the Intergovernmental Panel on Climate Change and the International Energy Agency, CCUS could capture a staggering 1 billion metric tons of CO₂ per year by 2030 and several billion tons by 2050. If carbon dioxide emissions from industrial and other sectors remain roughly the same when this capability is reached, it could reduce atmospheric greenhouse gas emissions by approximately 10%.
Insights
Models from international climate researchers predict the ability to capture nearly 10% of carbon dioxide gas that otherwise would have contributed to atmospheric GHG emissions by 2050.
CCUS technologies require reliable measurement at critical points to ensure process quality and safety. It typically includes level, flow, temperature, pressure, liquid analysis, and, increasingly, gas analysis, using Raman spectroscopic and TDLAS analyzers.
Given the high cost of capturing CO₂, the ability to transform significant volumes of this gas into a valuable product is of interest to processors implementing CCUS technologies. The applications and industries that can benefit from carbon utilization are numerous and include, but are not limited to, the following examples:
Better building materials for the construction industry
Known for its significant energy consumption and carbon footprint, the construction industry can leverage carbon capture and utilization to create more sustainable building materials. Traditional cement production processes heat materials to upwards of 1450°C (2642°F) generally using heavy fuel oil, coal, natural gas or other waste-derived fuels. Additionally, the chemical reaction typically used to make cement requires transforming calcium carbonate into calcium-oxide-like compounds, which generates CO₂ as a byproduct. Combined, these emissions are responsible for approximately 7% of world greenhouse gas production.
However, this can be mitigated by collecting carbon dioxide from the exhaust stream via amine gas treatment and injecting it into fresh concrete during the mixing process. The injected CO₂ reacts with calcium ions present in the concrete mix, forming calcium carbonate, a naturally occurring binding agent. This enriches the concrete by increasing its compressive strength and permanently sequestering the carbon dioxide, eliminating the need for storage and monitoring in geological formations.

This increased concrete strength enables material reductions in building projects, providing savings to partially offset the cost of amine treatment. Furthermore, CO₂ injection can be integrated into existing concrete production processes with minimal modifications to existing infrastructure.
Carbon dioxide can also be used to create synthetic aggregates, a key component of concrete, replacing traditional aggregates mined from the earth. Additionally, emerging innovators are exploring the development of bio-based carbon-negative alternatives to concrete, in which the production process absorbs more CO₂ than it emits.
Sustainable fuel for the transportation sector
The transportation sector, heavily reliant on fossil fuels, can reap significant sustainability benefits from carbon utilization. Through various chemical processes, captured CO₂ can be converted into renewable fuels like methanol and sustainable aviation fuel (SAF), helping reduce the industry’s carbon footprint.
To produce renewable methanol, captured carbon dioxide is reacted with green hydrogen in the presence of a catalyst at high temperature and pressure. Methanol can be used either as a direct fuel for automobiles or as a feedstock for other fuels, such as biodiesel.
S&P Global projects the renewable methanol market will reach 400 million metric tons annually by 2050, demonstrating its immense potential. However, there are challenges with using methanol in the transportation industry, including the need for specialized infrastructure.
Similarly, the aviation industry is exploring the use of SAF produced from captured CO₂ to reduce its reliance on fossil fuels. To make SAF, captured carbon dioxide is first combined with hydrogen in a process called reverse water-gas shift, creating syngas, a blend of carbon monoxide and hydrogen.
Next, the syngas is converted into a mixture of hydrocarbons using the Fischer-Tropsch process, and the hydrocarbon mixture undergoes hydroprocessing to remove impurities and adjust the fuel properties. Aviation fuel characteristics, including energy content, flash point and freezing point, must be carefully monitored and controlled.
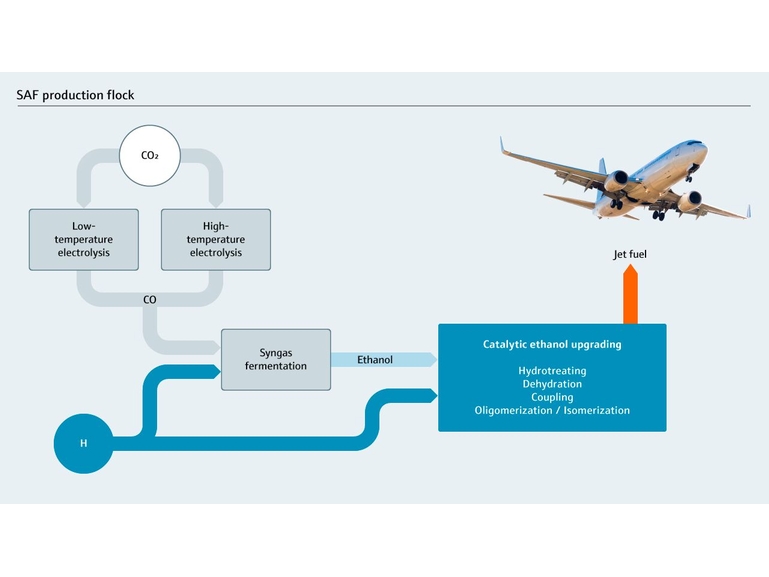
Insights
SAF is predicted to reduce GHG emissions in the aviation industry by up to 65% polymer production in the chemical industry once fully developed and implemented.
Polymer production in the chemical industry
The chemical industry currently relies heavily on fossil fuels, but carbon dioxide can be used as an alternative feedstock in many cases to produce a wide range of chemicals and polymers. These include fertilizer urea, plastics and packaging materials.
When CO₂ reacts with ammonia under high pressure and temperature, ammonium carbamate is formed. As this chemical decomposes, it produces urea and water, which can then be processed and granulated into a solid form for use as a fertilizer.
Carbon dioxide can also be used to produce polycarbonates. These durable and transparent polymers are commonly used in electronics, eyeglasses and automotive parts. These polymers are formed by directly reacting CO₂ with epoxides, a type of cyclic ether, in the presence of a catalyst.
Polyurethane production is another leading use for carbon dioxide in the chemical industry. Known for their versatility and applications in foams, coatings and insulation, polyurethanes are traditionally made using polyols derived from fossil fuels. However, processors can replace these with carbon dioxide-based polyols, reducing reliance on conventional fuels to lower polyurethane products’ carbon footprint.
These circular economy sustainable practices show promise, but they face challenges competing with traditional fossil fuel-based production due to the higher costs associated with carbon capture.
Improved food security in the agricultural sector
The agricultural sector can also benefit from carbon utilization through the application of urea fertilizers and direct use. Carbon dioxide gas can enrich greenhouse environments to enhance plant growth and improve yields. Moreover, captured CO₂ can be used to cultivate algae, which can be processed into biofuels, animal feed and food products.
A strong partnership for decarbonization
Endress+Hauser instrumentation helps ensure safe capture, purification and liquefaction of biogenic CO2. Learn more about the fruitful partnership with VERDEMOBIL BIOGAZ enabling reliable carbon capture on biomethane sites here.
Adopting CCUS
Despite its sustainable potential, CCUS adoption faces substantial obstacles. Carbon capture technologies, in particular, are costly to implement. Scaling up production to curtail GHG emissions will require significant investment in infrastructure and market development. Governments and non-governmental organizations will likely need to front a great deal of the necessary capital.
Furthermore, ensuring carbon capture and utilization’s overall sustainability requires powering the processes with energy derived from renewable sources. Using fossil fuels to power CCUS technologies would be counterproductive, negating the environmental benefits.
Over time, research and development will help optimize processes and enhance efficiency, decreasing the operational costs of CCUS technology. Economic viability, or at least low-cost carbon capture, is crucial for its widespread adoption throughout industry, especially as more companies and consumers globally value sustainability.
Further exploration in the carbon capture and utilization realm can help reduce GHG emissions and turn a harmful byproduct into a valuable resource. Carbon utilization provides one of many means to decrease carbon dioxide emissions across various industries, contributing to a more sustainable future.